During the early to mid 20th century, a broad range of antibiotics was discovered in rapid succession, being termed the golden age of antibiotics, though by the latter half of the century new drug discovery had slowed significantly. In part this was due to the rising tide of drug-resistant bacteria emerging around the world, first noted in Salmonella, Shigella, and Escherichia coli strains in the 1950s and 60s.
Before this time penicillin and other antibiotic drugs were available over the counter in the USA, and use had steadily been increasing in agriculture, resulting in several strains resistant to common drugs such as ampicillin.
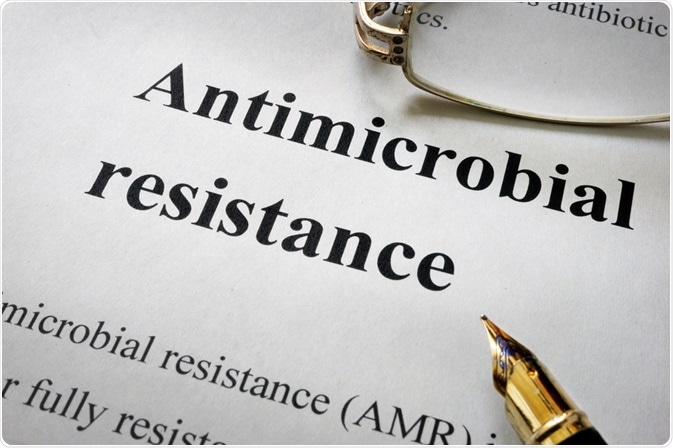
Image Credit: Vitalii Vodolazskyi/Shutterstock.com
The development of resistance is driven by natural selection pressures, and thus those bacterium with an advantage in surviving in the presence of toxins, antibiotics, will outcompete their neighbors. Common mechanisms of resistance include the development of efflux pumps that remove the drug from the cell following uptake, enzymes that disable the drug, adaptations to receptors that prevent interaction with the drug, and many more.
Exacerbating factors in the development of resistance include incomplete courses or insufficient doses of antibiotic medication, allowing bacteria that survive sub-lethal levels of drug exposure, and thus being more likely to already harbor some resistance mechanism, to propagate and become dominant.
Bacteria engage extensively in horizontal gene transfer, the exchange of genetic information between living, mature cells. This process can take place between pathogenic and non-pathogenic bacteria, allowing otherwise passive bacteria in the environment to act as a carrier or source of drug resistance genes for pathogenic strains.
Antibiotic pollution in the environment from both human waste and agricultural or industrial run-off is a major source of drug resistance, driven by low but persistent concentrations that quickly induce a positive selection pressure towards resistance. As much as 70% of an antibiotic may pass through humans unaltered to be excreted in the urine, passing into the environment where it enters the aquaculture and animals.
Even otherwise seemingly untouched environments have been found to harbor drug-resistant strains of bacteria: remote locations in water in the UK (Jones et al., 1986), in camp dogs in remote Australia (Rusdi et al., 2018), and Arctic birds (Sjölund et al., 2008).
The economic impact of drug resistance
Without new antibiotic treatments that evade resistance already developed, and preferably do not further induce the development of resistance, the global consequences will be extreme from clinical, social, and economic perspectives.
Already, patients suffering from multi-drug resistant infections cost the USA billions of dollars per year in treatment and prevention. Rare antibiotics used against resistant bacteria are extremely expensive, and those with resistant infections have hospital stays on average around twice as long as those without, also incurring further loss in terms of lost workdays. Subsequently, longer follow-up periods are required to treat these patients, and they are likely to remain out of work for some time, impacting familial finances.
In a recent report, the CDC stated that more than 2.8 million drug-resistant infections occur each year in the USA, with 35,000 dying. The six major drug-resistant strains: carbapenem-resistant Acinetobacter, carbapenem-resistant Enterobacteriaceae, extended-spectrum β-lactamase Enterobacteriaceae, multidrug-resistant Pseudomonas aeruginosa, methicillin-resistant Staphylococcus aureus, and vancomycin-resistant Enterococcus, alone are found to cost more than $4.6 billion annually, with many billions more lost in productivity.
In the report, the CDC identifies that infection prevention and control measures may have reduced probably overall infections by 18%, 30% in hospitals specifically, though that these efforts are still insufficient in the face of antibiotic resistance.
As has been seen during the coronavirus disease 2019 pandemic a significant outbreak of any disease could severely stall global trade and travel, though mortality rates from multi-drug resistant bacteria would likely far exceed that observed from SARS-CoV-2. In a 2019 review on the mortality rate of multidrug-resistant infections in India the overall rate was found to be 13.1%, 17.7% for Gram-negative species and 10.8% for Gram-positive, and rising to 26.9% and 16% for intensive care unit cases, respectively (Gandra et al., 2019).
Similarly, Santoro et al. (2020) found that almost 30% of over 1,000 blood infection patients bore multi-drug resistant strains, resulting in mortality rates of ~12%, 25%, and 36% at days 7, 30, and 90, respectively.
Potential solutions
Proper regulation of the use of antibiotics in humans and animals would limit the development of resistant strains, though unfortunately resistant strains are already here and spreading. Small molecule drugs appear to have been exhausted as a solution against multi-drug resistant bacteria, with the development of new antibiotics slowing notably in recent decades. Of the several dozen small molecule antibiotics currently under investigation by the FDA none tackle multi-drug resistant strains, and thus other solutions must be sought out.
Bio- and nano-technology-based approaches are under increasing scrutiny, utilizing proteins and engineered nanoparticles to either directly inhibit a specific pathogen or stimulate an immune response, potentially in the form of a vaccine. Nanomaterials in particular have several advantages over small molecule drugs with regards to biodistribution, retention time, and specificity, but also in avoiding the development of resistance in the first place.
Nanoparticles constructed from gold, silver, or copper, for example, exhibit antibacterial properties through the generation of reactive oxygen species and disruption of the bacterial cell membrane, against which resistance cannot develop, and such materials could be coated on wound dressings and surfaces to prevent infection.
References:
Further Reading